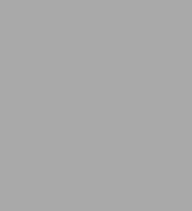
Single-particle cryoEM of Biological Macromolecules
120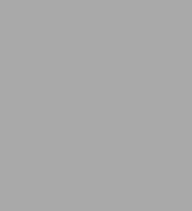
Single-particle cryoEM of Biological Macromolecules
120Hardcover
-
PICK UP IN STORECheck Availability at Nearby Stores
Available within 2 business hours
Related collections and offers
Overview
This edited book is written for students, postdocs and established investigators who want to enter the field of single-particle cryo-EM. This is a recently developed method to determine high-resolution structures of biological macromolecules. A major strength is the fact that cryo-EM does not require prior crystallization of protein complexes. It is especially well suited for larger complexes and molecular machines. This book, provides a comprehensive, accessible and authoritative introduction to the field. It covers all necessary background, ranging from the underlying concepts to practical aspects such as specimen preparation, data-collection, data analysis, and the final validation of results.
Key features
- Written for students, postdocs and established investigators who want to enter the field of single-particle cryo-EM
- Provides a comprehensive, accessible and authoritative introduction to the field of high-resolution structure analysis by single-article cryo-EM
- Covers all necessary background, ranging from the underlying concepts to practical aspects such as specimen preparation, data-collection, data analysis, and the final validation of results
- Authors of individual sections of this book have been recruited from among the most authoritative leaders in each topic
Product Details
ISBN-13: | 9780750330374 |
---|---|
Publisher: | Iop Publishing Ltd |
Publication date: | 08/31/2021 |
Series: | Biophysical Society |
Pages: | 120 |
Sales rank: | 1,059,017 |
Product dimensions: | 7.44(w) x 10.39(h) x 0.91(d) |
About the Author
Professor Nogales (UC Berkeley) has used cryo-EM throughout her scientific career, successfully applying this technique for the last three decades that defied structural characterization.
Professor Chiu (Stanford University) developed early experimental and computational methods towards 3D structure determination of biomolecules towards 3D atomic resolution: contributed to the establishment of validation methods to assess cryoEM structures.
Table of Contents
Editor biographies xv
Section authors xvi
1 Introduction and overview 1-1
1.1 Visualizing biological molecules to understand life's principles 1-1
1.1.1 A brief historical perspective on scattering-based structural biology methods 1-1
1.1.2 Unique capabilities of cryo-EM: polymers and viruses 1-3
1.1.3 Unique capabilities of cryo-EM: integral membrane proteins 1-4
1.1.4 Unique capabilities of cryo-EM: large assemblies 1-5
1.1.5 Unique capabilities of cryo-EM: scarce samples 1-5
1.1.6 Unique capabilities of cryo-EM: compositionally heterogeneous samples 1-6
1.1.7 Unique capabilities of cryo-EM: conformationally complex samples 1-7
1.1.8 Current limits of cryo-EM and things yet to come 1-8
1.2 Recovery of 3D structures from images of weak-phase objects 1-9
1.2.1 The signal that we care about is attributed to elastic scattering of electrons 1-10
1.2.2 The electron accumulates information as it passes through a specimen 1-10
1.2.3 The image wave function, and thus the image intensity, suffers from imperfections in the microscope optics 1-12
1.2.4 Intermediate summary: the image intensity is linear in the projected Coulomb potential of the object 1-14
1.2.5 Structure-factor phases, as well as amplitudes, are retained in the computed Fourier transforms of image intensities 1-15
1.2.6 The projection theorem: the Fourier transform of an image corresponds to a 2D 'central' section within the 3D Fourier transform of the object 1-17
1.2.7 The 3D object can be reconstructed from multiple projections 1-18
1.2.8 Similarities and differences between sub-tomogram averaging and single-particle cryo-EM 1-19
References 1-20
2 Sample preparation 2-1
2.1 Overview 2-1
2.2 Initial screening of samples in negative stain 2-2
2.2.1 Introduction 2-2
2.2.2 Negative staining for TEM 2-2
2.2.3 Purpose of negative staining when starting a project 2-3
2.2.4 Techniques for the preparation of negatively stained samples 2-4
2.2.5 Use of data processing to provide feedback to optimize samples for cryo-EM 2-10
2.3 Standard method of making grids for cryo-EM 2-13
2.3.1 Grids and support films 2-14
2.3.2 Plasma cleaning or 'glow discharging' grids 2-15
2.3.3 Types of apparatus used for plunge freezing 2-17
2.3.4 Blotting and plunging the grid using plunge freezers 2-18
2.3.5 Common issues faced in making grids for cryo-EM imaging 2-19
2.4 Requirement to make very thin specimens for cryo-EM 2-19
2.4.1 Inelastic electron scattering causes the image quality to deteriorate with increasing sample thickness values 2-20
2.4.2 The projection approximation may fail if the sample is too thick 2-21
2.4.3 Areas of a grid where the sample is obviously too thick can, and should be, avoided during data collection 2-21
2.4.4 Areas where the sample is much too thin, perhaps even air-dried, can sometimes be avoided just on the basis of their subjective appearance 2-23
2.5 Current strategies for optimizing preparation of cryo-grids 2-25
2.5.1 Behavior of particles in the thin film environment 2-25
2.5.2 Approaches to alter particle behavior in the thin film 2-28
2.5.3 New technologies for sample preparation 2-32
References 2-37
3 Data collection 3-1
3.1 Overview 3-1
3.2 Radiation damage in cryo-EM 3-2
3.2.1 Introduction 3-2
3.2.2 Interaction cross sections, elastic, and inelastic interactions 3-2
3.2.3 Cryoprotection and primary, secondary, and tertiary radiation damage 3-3
3.2.4 Radiation damage dependence on electron energy 3-4
3.2.5 Practical implications of radiation damage: image averaging in cryo-EM 3-5
3.2.6 Resolution dependence and exposure weighting 3-6
3.2.7 Radiation damage versus beam-induced motion and charging 3-9
3.3 Low-dose protocols for recording images 3-10
3.3.1 Automated low-dose imaging 3-10
3.3.2 Improving throughput 3-13
3.3.3 Electron exposure levels used during high-resolution data collection 3-15
3.4 Practical considerations: defocus. stigmation, coma-free illumination, and phase plates 3-15
3.4.1 Why do we need to defocus the microscope? 3-15
3.4.2 Effects of defocus on the image and its information content 3-16
3.4.3 Defocus variation is necessary to obtain uniform information coverage in reciprocal space 3-17
3.4.4 Optical correction of astigmatism and coma aberrations 3-19
3.4.5 Use of phase plates to improve image contrast and the expected benefits 3-20
3.5 Practical considerations: movie-mode data acquisition 3-23
3.5.1 Magnification and resolution 3-24
3.5.2 Dose rate 3-25
3.5.3 Strategies for motion correction 3-26
3.5.4 Total dose or exposure time 3-29
3.5.5 File size of movie datasets 3-29
3.5.6 Summary 3-29
References 3-30
4 Data processing 4-1
4.1 Overview 4-1
4.2 Automated extraction of particles 4-2
4.2.1 From micrographs to particles 4-2
4.2.2 Manual selection 4-3
4.2.3 Unbiased automated approaches 4-4
4.2.4 Particle extraction 4-8
4.2.5 Cleaning up the results through classification 4-9
4.3 CTF estimation and image correction (restoration) 4-10
4.3.1 CTF estimation 4-13
4.3.2 Image correction 4-16
4.3.3 Magnification distortion 4-18
4.3.4 Concluding remarks 4-20
4.4 Merging data from structurally homogeneous subsets 4-20
4.4.1 How many particle images are needed for a 3D reconstruction? 4-21
4.4.2 Obtaining a 3D reconstruction 4-28
Acknowledgments 4-37
4.5 3D classification of structurally heterogeneous particles 4-37
4.5.1 Introduction 4-37
4.5.2 Global 3D classification 4-38
4.5.3 Masked 3D classification 4-40
4.5.4 3D classification of particles with pseudo-symmetry 4-43
4.5.5 Dealing with continuous motions 4-44
4.5.6 Conclusion 4-45
4.6 Preferred orientation: how to recognize and deal with adverse effects 4-45
4.6.1 Protein interaction with the air-water interface 4-46
4.6.2 Preferred orientation and its effects in cryo-EM 4-47
4.6.3 Quantifying preferred orientation and its effects on cryo-EM reconstructions 4-50
4.6.4 Overcoming the effects of preferred orientation 4-53
4.6.5 Areas of research 4-57
Acknowledgments 4-59
4.7 B factors and map sharpening 4-59
4.7.1 An ideal 3D reconstruction has a predictable radial amplitude spectrum 4-59
4.7.2 Actual 3D reconstructions feature dampened amplitudes at high frequencies 4-59
4.7.3 Several factors contribute to signal decay at high frequencies 4-62
4.7.4 Gaussian falloff, parametrized by a B factor, is a useful model of signal loss 4-62
4.7.5 Estimating B factors 4-63
4.7.6 Sharpening a map 4-64
4.7.7 A single inverse Gaussian filter using a global B factor does not always lead to the optimal map 4-65
4.8 Optical aberrations and Ewald sphere curvature 4-67
4.8.1 Further considerations on the aberration function γ(s) 4-68
4.8.2 Common types of aberrations 4-70
4.8.3 Practical considerations for aberration correction 4-72
4.8.4 Thick objects and the Ewald sphere 4-76
4.8.5 Ewald sphere correction 4-77
References 4-80
5 Map validation 5-1
5.1 Overview 5-1
5.2 Measures of resolution: FSC and local resolution 5-2
5.2.1 The 'gold-standard' FSC 5-5
5.2.2 Resolution thresholds 5-5
5.2.3 FSC artifacts due to masking, filtration, and CTF 5-6
5.2.4 Local resolution 5-8
5.2.5 Resolution anisotropy 5-10
5.3 Recognizing the effect of bias and over-fitting 5-10
5.3.1 Introduction and nature of the problem arising from iterative refinement 5-10
5.3.2 Assessing the consistency of maps with projection data 5-12
5.3.3 Detecting over-fitting at high resolution in maps and effect on the FSC 5-15
5.3.4 Local over-fitting 5-18
5.3.5 Conclusion 5-21
5.4 Estimates of alignment accuracy 5-21
5.4.1 Correlation and the signal-to-noise ratio (SNR) 5-22
5.4.2 Analysis of alignment accuracy with synthetic data 5-23
5.4.3 The relationship between alignment accuracy and resolution 5-25
5.4.4 Estimating alignment accuracy from tilt pairs 5-27
5.4.5 Estimating alignment accuracy from the reconstructed map 5-27
5.4.6 Estimating alignment accuracy from projection-matching results 5-28
5.5 Discussion 5-28
Acknowledgements 5-29
References 5-29
6 Model building and validation 6-1
6.1 Overview 6-1
6.2 Using known components or homologs: model building 6-2
6.2.1 Identifying known/modeled structures of individual subunits 6-3
6.2.2 Rigid-body fitting 6-4
6.2.3 Flexible fitting 6-4
6.3 Building atomistic models in cryo-EM density maps 6-6
6.3.1 Introduction 6-6
6.3.2 Building models into cryo-EM density maps 6-10
6.3.3 Model refinement 6-15
6.3.4 Model validation 6-17
6.3.5 Model uncertainty 6-20
6.3.6 Model deposition 6-21
6.3.7 Revisiting the cryo-EM model challenge 6-22
6.3.8 Toward the future 6-22
6.3.9 Conclusions 6-25
Acknowledgments 6-25
6.4 Quality evaluation of cryo-EM map-derived models 6-26
6.4.1 Introduction 6-26
6.4.2 Map-model metrics 6-27
6.4.3 Model-only metrics 6-37
6.4.4 Summary and conclusions 6-39
Acknowledgment 6-40
6.5 How algorithms from crystallography are helping electron cryo-microscopy 6-40
6.5.1 Introduction 6-40
6.5.2 Map improvement 6-42
6.5.3 Map interpretation and model building 6-45
6.5.4 Model optimization 6-48
6.5.5 Validation 6-50
6.5.6 Validation-guided corrections 6-52
6.5.7 Conclusions 6-54
Acknowledgments 6-54
6.6 Archiving structures and data 6-55
6.6.1 Introduction 6-55
6.6.2 Single-particle cryo-EM structure deposition 6-56
6.6.3 Preparing files for deposition 6-57
6.6.4 Data validation 6-59
6.6.5 Sample sequence and ligands 6-61
6.6.6 Deposition using OneDep 6-61
6.6.7 Post-deposition: what happens next? 6-64
6.6.8 Accessing cryo-EM structure data 6-65
6.6.9 Conclusions 6-66
Acknowledgment 6-66
References 6-66